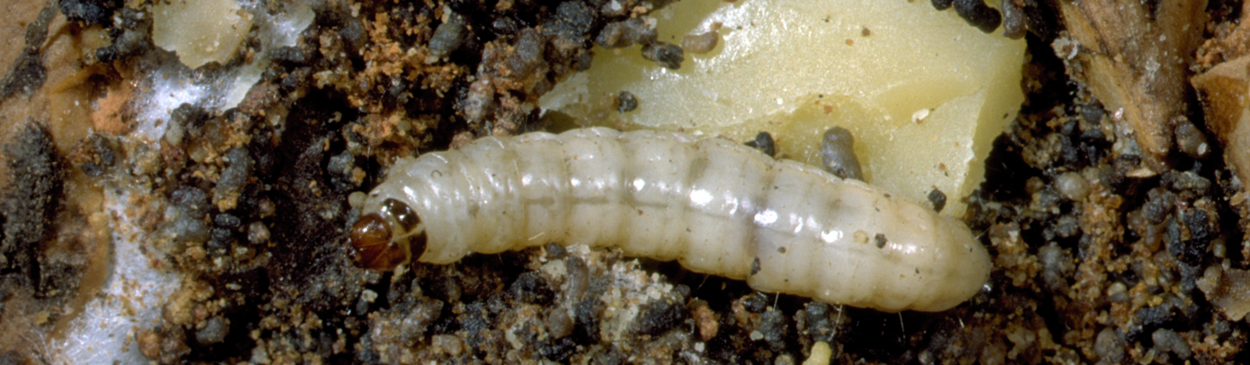
Some of the biological products used in conventionally or organically produced nut crops include those based on bacteria (abamectin, Bacillus amyloliquefaciens, Bacillus mycoides, Bacillus thuringiensis (Bt,) Burkholderia rinojensis, Chromobacterium subtsugae and spinosad,) fungi (Isaria fumosorosea, Paecilomyces lilacinus,) virus (Cydia pomonella granulovirus) and botanicals (azadirachtin, neem oil, pyrethrins, and some essential oils.)Â Except for B. amyloliquefaciens and B. mycoides for disease control and P. lilacinus for nematode control, the rest of the products are used for controlling insect and mite pests. While bacteria and the virus are primarily used against lepidopterous pests, others are used for insect and/or mite pests. Research shows that entomopathogenic nematodes such as Steinernema carpocapsae and Steinernema felitiae can also be effectively used against the navel orangeworm (Amyelois transitella.) There are other biological products that can be used against one or more pests of nut crops depending on the cost of the treatment.
Modes of Action
When using biological pesticides, understanding their mode of action or infection is very important. For example, Bt needs to be ingested by the target insect and hence is ideal for lepidopteran larvae which has chewing mouthparts. Viruses also need to be ingested by the insect and are generally specific to a particular species of insect or a closely related species. Both bacteria and viruses act in the intestines of the target insects.
Entomopathogenic fungi such as Isaria fumosorosea cause infection through contact and can be used against a wide variety of pests. Infective juveniles of entomopathogenic nematodes seek out their hosts, enter the host body through natural orifices, and the symbiotic bacteria in the nematodes kill the host insect. Depending on the active ingredient, botanical pesticides have different modes of action. Azadirachtin, for example, is an insect repellent, antifeedant, growth regulator and insecticide. While pyrethrins are nerve poisons, oil-based pesticides and their toxic compounds can suffocate, repel, or kill target arthropods through one or more modes.
Abamectin, spinosad and products containing the metabolites of B. rinojensis and C. subtsugae derive their activity from their toxic molecules. Both abamectin and spinosad are nerve poisons, but the other two bacterial metabolites have complex modes of action resulting in arthropod mortality or repellence. It is important to understand the biology of the target pest, its vulnerable stages, the suitability of various biopesticides to the target life stages of the pest, and then using them according to the label guidelines will ensure the success of biopesticides. Some of the biopesticides contain live organisms, so proper storage, transportation and mixing with compatible materials during application are critical for their efficacy.
Whether it is a synthetic or biological pesticide, there are certain basic principles for their effective use. Applying according to the label guidelines, avoiding excessive and repetitive use of any particular pesticide or those with the same mode of action, and alternating or rotating pesticides among multiple modes of action are essential for maintaining pest control efficacy and reducing the risk of resistance development. IPM principles are to be followed in both conventional and organic production systems where pesticides are encouraged to be used only as needed after exploring all non-pesticidal options.
Botanical Pesticides and Resistance
Repeated use of any pesticide can create selection pressure on arthropod populations, and those with beneficial mutations will resist both synthetic and biological pesticides. Arthropods develop resistance to pesticides through genetic, metabolic, or behavioral changes resulting in reduced penetration of toxin, increased sequestration or excretion, reduced binding to the target site, altered target site that prevents binding of the toxin, or reduced exposure to the toxin through modified behavior. Depending on the mode of action, both synthetic and biological pesticides can lead to resistance problems from excessive use.
Botanical insecticide pyrethrum, extracted from the flowers of Chrysanthemum cinerariaefolium and C. cineum, contains insecticidal esters known as the pyrethrins. Pyrethrins are nerve poisons disrupting the sodium channels in neurotransmission and are commonly used for controlling pests in agricultural, structural, and public and animal health sectors. Arthropod resistance to pyrethrins and their synthetic analogs, pyrethroids, is very common and has been reported for several decades. Insect mutations that reduce the penetration of the toxin through the cuticle, reduce its binding to the target site, or alter the properties of the sodium channels, and other mechanisms impart pyrethrin resistance. The navel orangeworm is one of the examples that developed pyrethrin resistance.
Another botanical insecticidal compound, azadirachtin, is a tetranortriterpenoid limonoid from neem (Azadirachta indica) seeds, which acts as an insecticide, antifeedant, repellent, and insect growth regulator. While neem oil, which has a lower concentration of azadirachtin, has been used in the US as a fungicide, acaricide and insecticide for a long time, several azadirachtin formulations in powder and liquid forms have become popular in recent years.
While there was a report of artificially induced resistance to azadirachtin in the green peach aphid, several studies demonstrated the efficacy of azadirachtin against various pests and recommended it as an option in IPM and for managing resistance to other pesticides. Azadirachtin is also thought to reduce the production of detoxification enzymes and known to improve the efficacy of other biopesticides. While arthropod resistance to botanical pesticides other than pyrethrins is not commonly reported, it is known that plant allelochemicals (e.g. alkaloids, phenolics, terpenoids, etc.) can contribute to cross-resistance to certain chemical pesticides. It is important to monitor the potential risk of resistance development and cross-resistance when botanical pesticides are used.
Bacterial Biopesticides and Resistance
Abamectin is derived from the bacterium Streptomyces avermitilis and contains insecticidal and nematocidal avermectins (avermectin B1a and B1b) which are nerve poisons. Abamectin interferes with neuromuscular transmission and paralyzes the target pest. Resistant mite and insect populations have higher levels of detoxifying enzymes that sequester abamectin. Abamectin-resistant arthropods can develop cross-resistance to emamectin benzoate, spinosad and fipronil. The twospotted spider mite, diamondback moth, Colorado potato beetle and vegetable leafminer are some examples of resistant arthropods to abamectin.
Bt, which contains crystalline toxic protein that is activated upon ingestion by an insect host, is a gram-positive soil bacterium that binds to the receptor sites in the midgut, and eventually causes insect death. Bt pesticides are used against a variety of lepidopteran (Bt subsp. aizawai and Bt subsp. kurstaki,) dipteran (Bt subsp. israelensis and Bt subsp. sphaericus) and coleopteran (Bt subsp. tenebrionis) pests. Since the mode of action involves toxins rather than the bacterial infection itself, resistance to Bt pesticides or transgenic crops that contain Bt toxins is also very common. Some examples of resistant insects to Bt include the beet armyworm, cabbage looper, corn earworm, Colorado potato beetle and diamondback moth. Mutations in insects that regulate the immune system or interfere with the activation of Bt toxins and their binding to the target site led to insect resistance.
Spinosad is a mixture of macrocyclic lactones, spinosyns A and spinosyns D, derived from Saccharopolyspora spinosa, an actinomycete gram-positive bacterium, and is used against dipteran, hymenopteran, lepidopteran, thysanopteran and other pests. Although naturally derived, some spinosad products are not registered as biopesticides.
Insect resistance to spinosad later led to the development of spinetoram, which is a mixture of chemically modified spinosyns J and L. Both spinosad and spinetoram are contact and stomach poisons and act on insect nervous system by continuous activation of nicotinic acetylcholine receptors. The American serpentine leafminer, beet armyworm, diamondback moth, tomato borer, onion thrips, and western flower thrips are some insects with spinosad resistance. Production of detoxifying enzymes (metabolic resistance) and changes in the target site are the most common mechanisms of spinosad resistance in insects. Cross-resistance between spinosad and some chemical insecticides has also occurred in some insects.
Viral Biopesticides and Resistance
Baculovirus infections in Lepidoptera have been known for centuries, especially in silkworms. Currently, there are several commercial formulations of nucleopolyhedroviruses (NPV) and granuloviruses (GV.) When virus particles are ingested by the insect host, usually a lepidopteran insect, they invade the nuclei of midgut, fatbody, or other tissue cells and kill the host. Baculoviruses are generally very specific to their host insect species and can be very effective in bringing down the pest populations. However, variations in the susceptibility of certain insect populations and development of resistance to viruses has occurred in several host species. Mutations in one or more genes with complex mechanisms are involved in insect resistance to viruses.
Fungal Biopesticides and Resistance
There are several fungi that infect insects and mites. The fungal infection starts when fungal spores come in contact with an arthropod host. First, they germinate and gain entry into the body by breaching through the cuticle. Fungus later multiplies, invades the host tissues, kills the host and emerges from the cadaver to produce more spores.
Entomophthoralean fungi such as Entomophthora spp., Pandora spp. and Neozygites spp. can be very effective in pest management through natural epizootics, but cannot be cultured in vitro for commercial-scale production. Hypocrealean fungi such as Beauveria bassiana, Isarea fumosorosea, Metarhizium brunneum and Verticillium lecanii, on the other hand, can be mass-produced in vitro and are commercially available. These fungi are comparable to broad-spectrum insecticides and are pathogenic to a variety of soil, foliar and fruit pests of several major orders. Since botanical, bacterial and viral biopesticides have insecticidal metabolites, proteins, or viral particles that have specific target sites and modes of action, insects have a higher chance of developing resistance through one or more mechanisms.
Although fungi also have insecticidal proteins such as beauvericin in B. bassiana and I. fumosorosea and dextruxin in M. anisopliae and M. brunneum, their mode of action is more through fungal infection and multiplication, and arthropods are less prone to developing resistance to entomopathogenic fungi. However, insects can develop resistance to entomopathogenic fungi through increased melanism, phenoloxidase activity, protease inhibitor production, and antimicrobial and antifungal peptide production. It also appears that the production of detoxification enzymes in insects against fungal infections can also impart resistance to chemical pesticides.
Good agricultural practices based on IPM principles help maintain pest control efficacy and reduce the risk of resistance development. Biopesticides are a critical part of IPM and are especially important in organic farming. A good understanding of biopesticides can help with their long-term use by avoiding resistance issues.